The Higgs Field
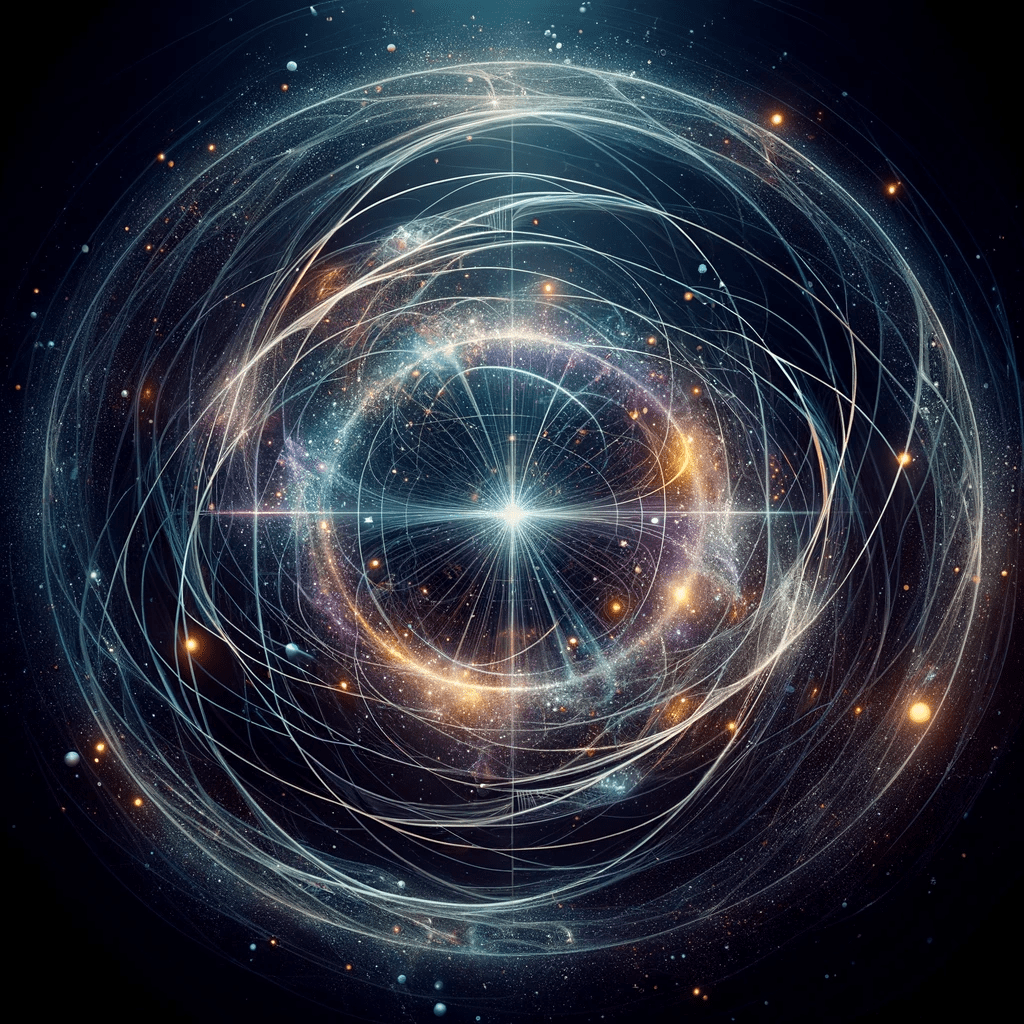
The Higgs field, a concept in particle physics, is responsible for giving particles mass, and was first proposed in the 1960s by physicist Peter Higgs. This groundbreaking idea has since been confirmed through experimental evidence and transformed our understanding of the universe. The Higgs field is an energy field that permeates all of space, and its effects are observed through the Higgs boson, a particle associated with this field. Interactions between particles and the Higgs field are responsible for endowing particles with mass, which is a fundamental property of matter. This discovery has had profound implications for our understanding of the universe’s formation and evolution, as well as the laws governing particle interactions.
In 2012, the discovery of the Higgs boson at the Large Hadron Collider (LHC) in CERN, Switzerland, provided experimental evidence to support the existence of the Higgs field. This marked a significant milestone in the field of particle physics, confirming the predictions made by the Standard Model, which describes the behavior of subatomic particles and the forces that act on them. The discovery of the Higgs boson was awarded the Nobel Prize in Physics in 2013, recognizing its importance in advancing our understanding of the universe.
Fact 1: The Higgs field is a scalar field with a non-zero vacuum expectation value, meaning it has a constant value throughout space even in the absence of particles. This property distinguishes it from other fields and is responsible for giving particles mass (Source: CERN).
Fact 2: The discovery of the Higgs boson at the LHC in 2012 was the result of the collaborative efforts of thousands of scientists and engineers from around the world, who worked together on the ATLAS and CMS experiments (Source: CERN).
Fact 3: The Higgs mechanism, which describes how particles acquire mass through their interaction with the Higgs field, was independently proposed in 1964 by several groups of physicists, including Peter Higgs, François Englert and Robert Brout, and Gerald Guralnik, Carl Hagen, and Tom Kibble (Source: CERN).
Experts in the field of particle physics, such as Peter Higgs, François Englert, and John Ellis, have emphasized the importance of the Higgs field in understanding the fundamental properties of the universe. In their work, they explain that the Higgs field is essential for explaining the mass of particles and the forces that govern their interactions.
Popular science books like “The Particle at the End of the Universe” by Sean Carroll look into the discovery of the Higgs boson and the implications of the Higgs field for our understanding of the universe. These books aim to communicate the significance of this groundbreaking discovery to a broader audience, highlighting the elegance and ingenuity of the underlying theory.
It is possible for a particle to have no mass. The most well-known example of a massless particle is the photon, which is the carrier of the electromagnetic force and a quantum of electromagnetic radiation, such as light. Photons travel at the speed of light in a vacuum, and according to the theory of relativity, massless particles must always travel at this speed.
The fact that photons have no mass has significant implications for their behavior and the properties of electromagnetic radiation. For instance, it allows light to propagate over long distances without losing energy. In the context of the Higgs field, massless particles do not interact with the field, and thus, their lack of mass is a direct result of not interacting with the Higgs field.
Besides photons, another example of a massless particle is the gluon. Gluons are elementary particles that transmit the strong nuclear force, one of the four fundamental forces in nature. They are responsible for holding quarks together within protons, neutrons, and other hadrons.
Like photons, gluons are considered to be massless, although they play a crucial role in the interactions between particles that possess mass. However, it’s essential to note that gluons are always confined within hadrons due to a property called color confinement, which means that isolated gluons are not observed in nature.
It’s worth mentioning that neutrinos, another class of elementary particles, were once believed to be massless. However, the discovery of neutrino oscillations, which earned the 2015 Nobel Prize in Physics, provided evidence that neutrinos do have a small but nonzero mass. While their masses are tiny compared to other particles, they are not considered massless.
Manipulating the Higgs field directly is currently beyond our technological capabilities. The Higgs field is a fundamental aspect of the universe, and its influence on particles is a natural consequence of their interactions with the field. At present, we don’t possess the technology or knowledge required to control or manipulate the Higgs field.
However, scientists have been able to generate and study the Higgs boson, the particle associated with the Higgs field, using particle accelerators like the Large Hadron Collider (LHC) at CERN. By smashing high-energy protons together, they can create short-lived Higgs bosons and study their properties and interactions. These experiments have provided invaluable insights into the nature of the Higgs field and its role in particle physics. Although these experiments do not constitute direct manipulation of the Higgs field, they demonstrate our ability to probe the field’s effects indirectly.
In the future, advances in our understanding of the Higgs field and particle physics could potentially lead to new ways of interacting with or manipulating the Higgs field. However, such possibilities remain speculative and would likely require breakthroughs in both theoretical understanding and technological capabilities.