Terrahertz (THz) Waves
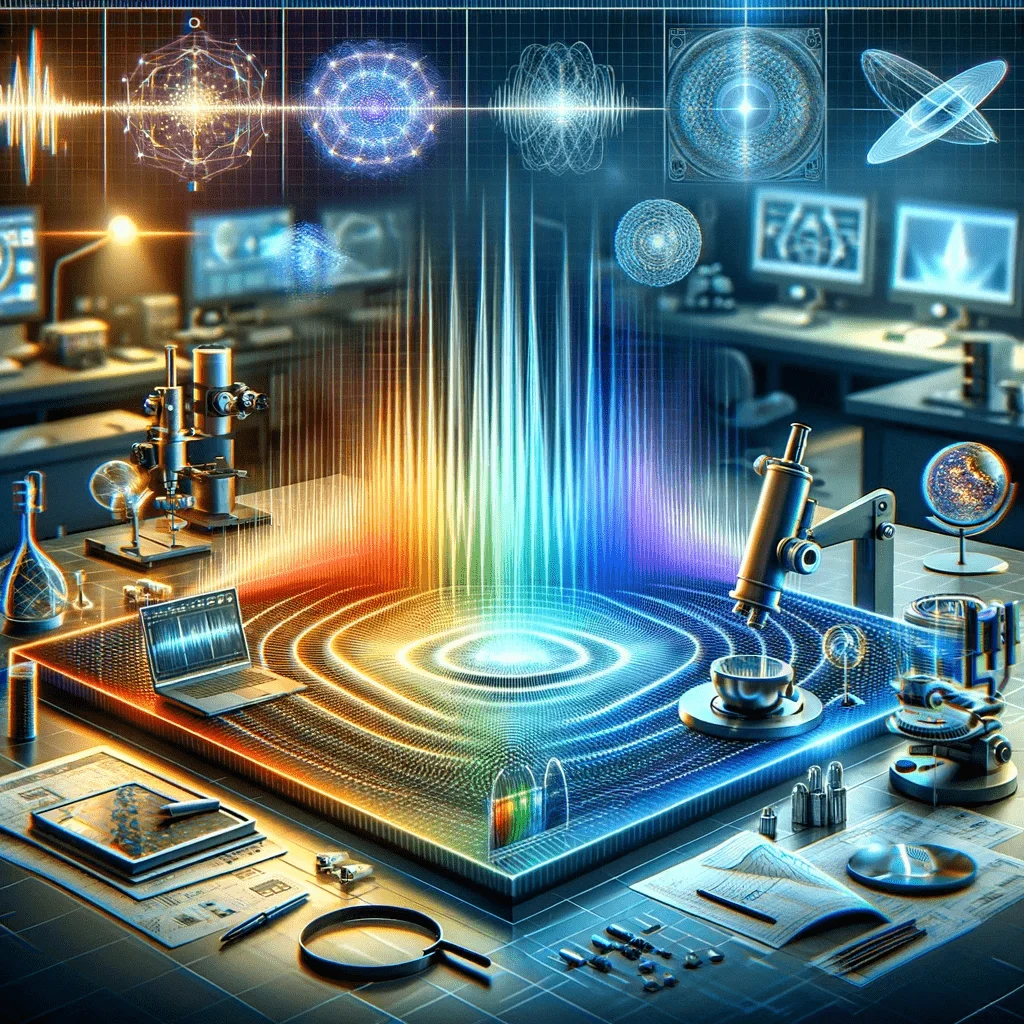
In an interview, Professor Garry Nolan discusses the use of terahertz waves in relation to a bismuth-magnesium material, which is associated with UAP phenomena. He notes that there were claims about the material levitating when subjected to terahertz waveforms. However, Nolan himself has not conducted this experiment because it requires a terahertz wave transmitter, which is a specialized and uncommon piece of equipment.
During the interview, Ross Coulthart asked Professor Garry Nolan a follow-up question about the terahertz wave research on the bismuth-magnesium material. In response, Nolan indicated that he couldn’t answer the question, implying that there might be restrictions or sensitivities regarding the information he could share publicly about this specific aspect of his research. This moment highlights the potential confidentiality or classified nature of some aspects of UAP-related research.
Terahertz (THz) waves, a type of electromagnetic radiation, sit in the spectrum between microwaves and infrared light, with frequencies ranging from 0.1 to 10 THz and corresponding wavelengths of 30 millimeters to 30 micrometers. These waves occupy the “terahertz gap,” a less explored part of the electromagnetic spectrum, where conventional electronic and optical methods face challenges in generating and detecting them.
Terahertz (THz) waves, like other forms of electromagnetic radiation outside the visible light spectrum, cannot be seen or heard by the human eyes or ears. Our sensory organs are limited to specific ranges of electromagnetic radiation and sound frequencies.
- Visibility: The human eye can detect only a small portion of the electromagnetic spectrum, known as visible light, which ranges from about 380 to 750 nanometers in wavelength. THz waves, with wavelengths ranging from 30 millimeters to 30 micrometers, are far outside this range. Therefore, they are invisible to the naked eye.
- Audibility: Similarly, the human ear can hear sounds in the frequency range of about 20 Hz to 20,000 Hz. THz waves, with frequencies between 0.1 and 10 THz, are many orders of magnitude higher than the highest frequency the human ear can detect. Consequently, THz waves are inaudible.
In summary, THz waves are neither visible nor audible to humans. They are detected and measured using specialized instruments and sensors designed for this purpose. One millimeter (mm) is equal to 1,000 micrometers (μm), and one micrometer is equal to 1,000 nanometers (nm).
Generating and detecting THz waves requires specialized techniques and equipment. THz time-domain spectroscopy (TDS), quantum cascade lasers, and photomixing sources are some methods used for generation, while detection can be achieved through methods like time-domain spectroscopy, bolometers, and Golay cells.
These technologies exploit the unique properties of THz waves to create images, analyze materials, and explore biological structures.
- Time-Domain Spectroscopy (TDS): This is a common method for detecting THz waves. In THz-TDS imaging systems, photoconductive antennas or nonlinear optical processes are used to generate and detect these waves. The process involves splitting an optical beam from a femtosecond laser into pump and probe branches. The pump pulses generate THz pulses which illuminate the object being imaged. The reflected or transmitted THz pulses are then detected, carrying information about the object. By varying the time delay between the pump and probe beams, the time-domain electric field profile of the THz signal is obtained, providing ultrafast temporal and spectral information about the imaged object.
- Frequency-Domain Terahertz Imaging: This type of imaging resolves only the intensity response of the imaged object. Coherent terahertz imaging can be achieved using a heterodyne detection scheme, which involves mixing the received radiation with a local oscillator beam and down-converting the THz frequency to a radio frequency. This allows for the use of high-performance RF electronics for signal detection. Detectors such as superconductor-insulator-superconductor (SIS) and hot-electron bolometer (HEB) mixers offer quantum-level sensitivities but require cryogenic cooling.
- Backward-Wave Oscillator (BWO): This is an important source for THz waves based on up-conversion, extending the frequency of microwave sources to the THz range using harmonic generation. BWOs are tunable and operate similarly to a traveling wave amplifier.
- Quantum Cascade Laser (QCL): These are semiconductor-based THz sources. QCLs use coupled quantum wells to split the conduction band into distinct sub-bands, creating population inversion between two sub-bands and enabling the generation of THz radiation.
- Stimulated Terahertz Amplitude Radiation (STAR) Emitters: These are solid-state emitters based on superconducting devices. They generate coherent electromagnetic waves at THz frequencies due to the Josephson effect between superconductor-insulator-superconductor (SIS) junctions.
- Photoconductive Antenna (PCA): Commonly used in THz TDS systems, a PCA consists of a semiconductor substrate with metallic electrodes, where photocarriers are generated upon illumination.
- Specialized Radar Systems: Advanced radar technologies have been developed that utilize THz waves for applications like rotating target detection. These systems manipulate the frequency to access different resonances in the beam emitter cavity and can accurately quantify the rotational speed of objects.
Each of these technologies is designed to exploit specific aspects of THz waves, such as their ability to penetrate non-conducting materials and their non-ionizing nature, making them suitable for a wide range of applications, from medical imaging to material analysis and security screening.
The applications of THz waves are diverse and growing, despite these challenges. In imaging and security, THz waves can penetrate materials without the harmful effects of X-rays, making them suitable for non-destructive evaluation and security screening. In spectroscopy, their unique ability to detect molecular absorption fingerprints in the THz range is useful for analyzing chemicals and materials. Additionally, in the realm of communications, THz waves offer the potential for high-speed wireless communications with bandwidths far exceeding those of current microwave frequencies.
The field of THz technology is a hotbed of active research, focusing on enhancing the generation, detection, and practical application of these waves. The significant potential of THz technology is continuously being unlocked through ongoing research, revealing new possibilities and applications across various fields.
It’s essential to recognize the existence of the so-called “terahertz gap,” a range in the THz region (0.1 to 10 THz) where practical technologies for generating and detecting radiation are still evolving. This gap arises due to the challenges in creating efficient power generation and receiver technologies within this range, especially for mass production and room temperature operation.
For instance, in 2008, engineers at Harvard University achieved room temperature emission of coherent THz radiation using a semiconductor source. This was a considerable development since previous sources required cryogenic cooling, limiting their practical applications. In 2013, researchers at the Georgia Institute of Technology and the Polytechnic University of Catalonia developed a method to create graphene antennas for emitting radio waves in the THz frequency range.
Terahertz waves are primarily known for their applications in imaging and spectroscopy, as they can penetrate various materials and provide detailed information about their composition. The concept of using electromagnetic waves for levitation typically involves different principles, such as magnetic levitation (maglev) using powerful magnets, or acoustic levitation using sound waves at certain frequencies. Not much is publicly known about using Terahertz (THz) waves for levitation.
The research on the effects of Terahertz (THz) waves on biomacromolecules is a fascinating area of study. Biomacromolecules, such as proteins, DNA, and RNA, play crucial roles in biological processes. These large molecules have specific structures that determine their function. The interaction of THz waves with these biomacromolecules can lead to changes in their structure and, consequently, their function. This means that THz waves can add energy to the molecules, causing them to rotate or vibrate differently. These changes in energy states can lead to alterations in the molecule’s spatial conformation, or its three-dimensional shape.
Audible Manifestations of UAP
While terahertz waves, due to their high-frequency nature, remain beyond the auditory threshold of human hearing, there have been numerous accounts of UAP encounters accompanied by unique auditory phenomena. These sounds, often described as buzzing, humming, or whirring noises, contrast with the silent operation typically expected from electromagnetic phenomena in the terahertz range.
The perception of sounds in UAP encounters could be attributed to various factors. It is possible that the mechanisms or technologies associated with UAPs, when interacting with the Earth’s atmosphere or materials, produce vibrations within the audible range of human hearing. Alternatively, these sounds could result from secondary effects, such as electromagnetic interference with devices or objects in the vicinity, translating electromagnetic energy into acoustic vibrations.
Notably, the auditory experiences associated with UAPs are as diverse as the visual sightings, suggesting a range of phenomena or technologies at play. Some witnesses report a sudden onset of sound that accompanies the visual appearance of a UAP, while others note a distinct sound that lingers after the object has vanished from sight. This discrepancy in reports highlights the complex nature of UAPs and the multifaceted ways in which they can interact with their surroundings.
Professor Garry Nolan’s insights into the research surrounding bismuth-magnesium materials, coupled with the technological challenges and opportunities presented by THz waves, underscore the vast, uncharted territories of electromagnetic science. The mysterious auditory phenomena associated with UAPs, seemingly at odds with the silent nature of THz waves, invite us to consider the broader implications of this research. It beckons us to remain open to the unknown, acknowledging the limitations of our sensory and technological capabilities, while continuously striving to expand the boundaries of knowledge and understanding in the quest to unravel the mysteries of UAP and the electromagnetic spectrum.