Quark-Gluon Plasma (QGP)
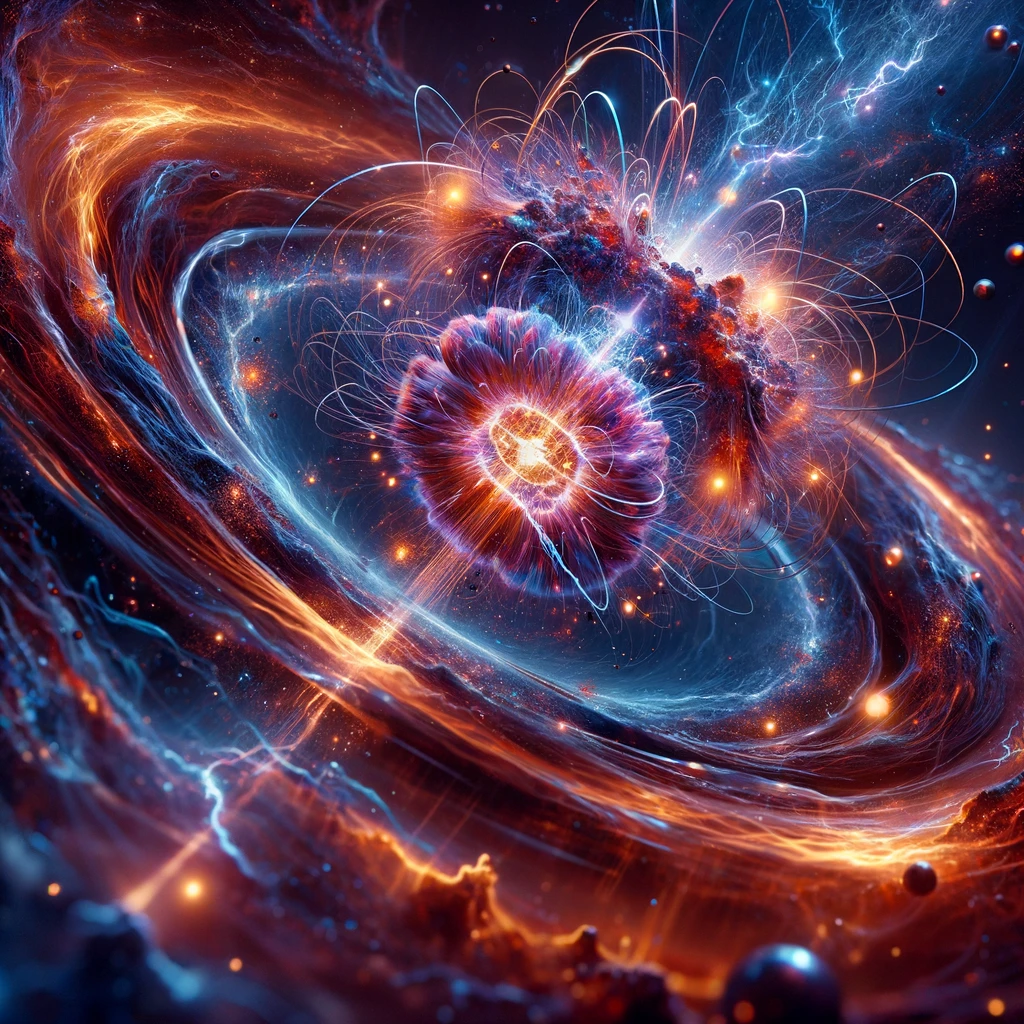
The exploration of quark-gluon plasma (QGP) through the STAR detector at the Relativistic Heavy Ion Collider has ushered in a new era of understanding matter under extreme conditions, akin to those in the early universe. This venture into the microscopic cosmos, where off-center collisions between heavy nuclei generate unprecedented magnetic fields, echoes the current leaps seen in science, particularly the reverse engineering of UFO and alien technologies. Just as advanced extraterrestrial civilizations inspire the pursuit of knowledge beyond our current technological grasp, the endeavor to decode the mysteries of QGP might be seen as a parallel quest in the realm of high-energy physics. The manipulation and study of such potent magnetic fields to probe the electrical conductivity of QGP could very well resemble the kind of advanced scientific understanding and technology one might associate with an advanced alien civilization.
The research using the STAR detector at the Relativistic Heavy Ion Collider has advanced our understanding of quark-gluon plasma (QGP), a form of matter created in extreme conditions during relativistic heavy-ion collisions. Notably, off-center collisions between heavy nuclei like gold or isobars such as ruthenium and zirconium produce very strong magnetic fields, estimated to be around 10 to the power of 18 gauss. These fields are used to investigate the electrical conductivity of the QGP.
These insights are vital for exploring the complex interactions and characteristics of this unique state of matter, reminiscent of the early universe’s conditions shortly after the Big Bang.
The energy involved in the exploration of quark-gluon plasma (QGP) through collisions in the Relativistic Heavy Ion Collider is immense and difficult to fathom in everyday terms. The magnetic fields generated in these collisions are around 10 to the power of 18 gauss, which is billions of times stronger than the magnetic field of Earth, which averages about 0.5 gauss. To put this into perspective, the strongest magnetic fields produced in a laboratory setting on Earth, outside of these high-energy physics experiments, are in the order of tens of thousands of gauss, and even these require sophisticated and powerful equipment.
The energy levels used in these experiments, such as 200 GeV (giga-electronvolts) and 27 GeV, are equally hard to conceptualize. One electronvolt is the amount of kinetic energy gained or lost by a single electron moving across an electric potential difference of one volt. A GeV is a billion electronvolts, indicating the particles are accelerated to incredibly high speeds, close to the speed of light. This energy is necessary to recreate the extreme conditions similar to those just after the Big Bang, allowing scientists to study the fundamental properties of matter at its most basic level.
In simpler terms, the energy and magnetic fields involved in these experiments are vastly beyond our everyday experience, akin to comparing the light of a candle to that of the sun. These experiments push the boundaries of our understanding of the universe, much like the exploration of advanced technologies would in the study of UFOs and alien civilizations.
When a Super Strong Magnetic Fields Leaves an Imprint on Nuclear Matter it refers to the impact of extremely powerful magnetic fields on the state and properties of nuclear matter, particularly during events known as heavy ion collisions. These collisions are studied to gain insights into the fundamental aspects of matter at the most basic level. New research findings offer new understanding about the electromagnetic characteristics of a specific state of matter called quark-gluon plasma (QGP).
Quark-Gluon Plasma (QGP) is a unique and extreme state of matter that is often compared to more familiar plasma states due to certain shared characteristics, but it also possesses distinct differences that set it apart.
Similarities with Other Plasma States:
- Ionized State: Like conventional plasmas, QGP is an ionized state of matter. In conventional plasma, atoms are ionized, meaning electrons are separated from their nuclei, leading to a soup of nuclei and electrons. In QGP, the ionization occurs at a more fundamental level, where quarks are not confined within protons and neutrons but are free to move in a sea of gluons.
- Conductivity: Both QGP and conventional plasmas are excellent conductors of electricity. The free charges in both states (electrons in conventional plasmas and quarks in QGP) allow them to conduct electrical current.
- Collective Behavior: Plasmas exhibit collective behavior due to long-range electromagnetic forces acting between charged particles. In QGP, although the forces involved are primarily the strong nuclear force (mediated by gluons), there is still a form of collective behavior among the quarks and gluons.
Differences from Other Plasma States:
- Forces Involved: Conventional plasmas are governed by electromagnetic forces between electrons and ions. In contrast, QGP is dominated by the strong nuclear force, which is much stronger than the electromagnetic force but acts over much shorter distances. This force is mediated by gluons, which bind quarks together.
- Temperature and Density: QGP exists at temperatures and densities that are several orders of magnitude higher than those found in conventional plasmas. The temperatures in QGP can reach over a trillion degrees Kelvin, conditions believed to be similar to those just after the Big Bang.
- Constituent Particles: While conventional plasmas consist of electrons and ions, QGP is composed of quarks and gluons, which are fundamental particles not found freely in nature under normal conditions.
- State of Matter: QGP is often referred to as the “fourth state of matter” beyond solids, liquids, and gases, with conventional plasma sometimes considered a subset of the gaseous state due to its ionized nature. However, QGP represents a further state beyond conventional plasma, often considered a separate, more extreme form of matter altogether.
While quark-gluon plasma shares some basic characteristics with other plasmas, such as being an ionized form of matter with high conductivity and collective behaviors, it is fundamentally different due to the particles involved, the forces at play, and the extreme conditions under which it exists. QGP provides a unique window into the conditions of the early universe and the behavior of matter under the most extreme conditions, distinguishing it from more familiar plasma states.
To break down this scientifically, let’s explore the key concepts involved:
- Super Strong Magnetic Fields: In the context of high-energy physics, super strong magnetic fields refer to magnetic fields that are significantly more intense than those we encounter in everyday life. In laboratories, these conditions are achieved during high-energy experiments, such as heavy ion collisions, where magnetic fields can reach magnitudes many times greater than anything naturally occurring on Earth.
- Imprint on Nuclear Matter: The term “imprint” suggests that the strong magnetic fields have a noticeable and measurable effect on the properties and behavior of nuclear matter, which is matter made up of protons and neutrons (collectively known as nucleons) and the interactions between them. The “imprint” could refer to changes in the state, structure, or dynamics of the nuclear matter due to the influence of the magnetic fields.
- Heavy Ion Collisions: These are experiments conducted using particle accelerators to smash together nuclei of heavy atoms (like gold or lead) at near-light speeds. The purpose of these collisions is to recreate conditions similar to those just after the Big Bang, allowing scientists to study the fundamental components and forces of matter.
- Electromagnetic Properties: This refers to the behavior of nuclear matter under the influence of electromagnetic forces, which include both electric and magnetic fields. These properties can reveal how charged particles within the nuclear matter, such as protons, electrons, and quarks, interact with each other and with external electromagnetic fields.
- Quark-Gluon Plasma (QGP): This is a state of matter believed to have existed just after the Big Bang, where quarks (the fundamental constituents of protons and neutrons) and gluons (the particles that mediate the strong force holding quarks together) are not confined within nucleons but exist as a free, hot, dense soup. Heavy ion collisions can momentarily create conditions hot and energetic enough to produce QGP, allowing scientists to study its properties.
Scientists have been exploring the phenomenon where off-center collisions of heavy atomic nuclei, like gold, produce exceptionally strong magnetic fields due to the motion of the non-colliding protons and neutrons as the ions graze each other at speeds near that of light. This interaction is thought to generate magnetic fields billions of times stronger than any found on Earth, potentially the strongest in the universe. However, the ephemeral nature of these fields, which dissipate in an unimaginably short span, makes them difficult to study directly. Instead, researchers at the Relativistic Heavy Ion Collider (RHIC) have used the STAR detector to observe the effects of these magnetic fields on the charged particles produced in the collisions. By examining the collective motion of these particles, scientists have detected patterns consistent with the influence of an electromagnetic field, indicative of Faraday induction within the quark-gluon plasma (QGP) created in these high-energy collisions. This discovery not only confirms the presence of these intense magnetic fields but also provides a novel method to investigate the QGP’s conductivity and its electromagnetic properties, offering new insights into fundamental physics and the behavior of matter under extreme conditions.
The sPHENIX detector represents a significant upgrade to the original PHENIX experiment at Brookhaven Lab’s Relativistic Heavy Ion Collider (RHIC), designed to enhance the study of quark-gluon plasma (QGP), a unique form of nuclear matter. This detector, comparable in size to a two-story building and weighing 1,000 tons, is equipped to capture 15,000 particle collision snapshots per second, significantly outpacing its predecessor, PHENIX. At its heart lies a cylindrical superconducting magnet, repurposed from the BaBar experiment at SLAC National Accelerator Laboratory, which plays a critical role in bending the trajectories of charged particles. This allows for the detailed analysis of particle interactions and the forces binding quarks and gluons. The sPHENIX’s advanced technology, including its inner and outer calorimeters, is key to measuring the energy and properties of particles resulting from collisions. This setup, combined with the superconducting solenoid magnet and high-acceptance calorimeters, provides the necessary speed and precision for tracking particle jets, heavy quarks, and other rare particles, offering insights into the QGP’s properties and the fundamental interactions of quarks and gluons.
Brookhaven National Laboratory stands as a beacon of scientific innovation and discovery, underpinned by its 2,500-plus dedicated staff members who spearhead and bolster a wide array of research endeavors. These efforts are crucial in advancing the mission of the Department of Energy (DOE) to safeguard the nation’s security and foster prosperity by tackling pressing energy, environmental, and nuclear challenges with cutting-edge science and technology solutions. The laboratory’s research portfolio is remarkably diverse, encompassing fields such as nuclear science, energy science, data science, particle physics, accelerator science and technology, quantitative plant science, and quantum information science. Brookhaven National Laboratory’s pivotal role is in pushing the boundaries of knowledge and innovation, supported primarily by the DOE’s Office of Science.
The direct connection between the study of QGP and the reverse engineering of extraterrestrial technology remains a conceptual leap required to understand and manipulate the universe at such a fundamental level shares a common spirit with the exploration of alien technologies. The research into QGP and its behaviors under extreme magnetic fields not only expands our understanding of the universe’s foundational building blocks but also embodies the human drive to explore, understand, and ultimately harness the laws of nature, much like the envisioned endeavors to decipher and utilize technologies from beyond our world.