Antimatter: A Fascinating Aspect of Particle Physics
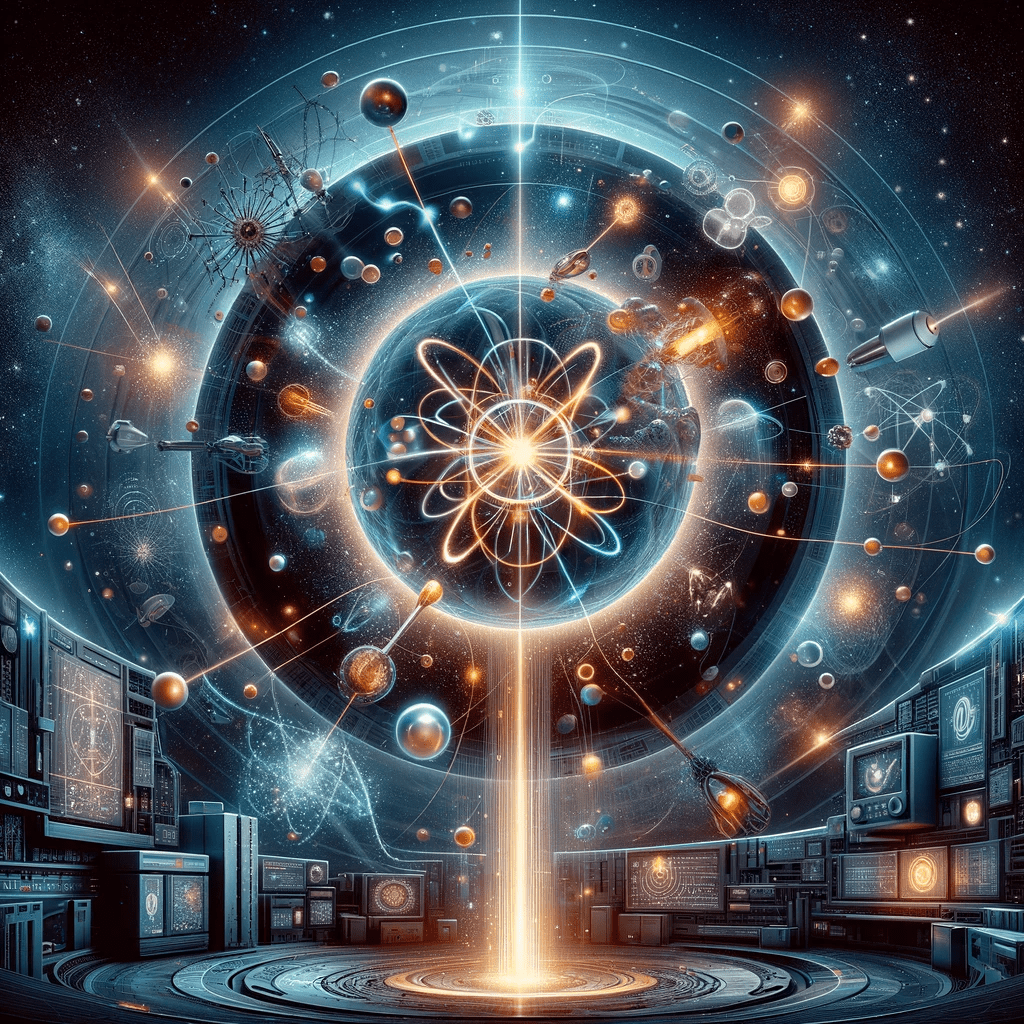
Antimatter is a fundamental aspect of particle physics that has captured the imagination of scientists and the public alike. This intriguing concept was first theorized by British physicist Paul Dirac in 1928, when he discovered that the equations of quantum mechanics allowed for the existence of particles with opposite properties to those of their normal matter counterparts. In essence, antimatter is a mirror image of ordinary matter, composed of antiparticles that have the same mass as their matter counterparts but opposite charge and other quantum properties. The study and understanding of antimatter are critical to advancing our knowledge of the universe, its origins, and the fundamental forces that govern it.
The existence of antimatter was experimentally confirmed in 1932 when American physicist Carl David Anderson discovered the positron, an antiparticle of the electron. Since then, researchers have been able to produce other forms of antimatter, such as antiprotons and antineutrons, in particle accelerators. Antiparticles and their matter counterparts annihilate each other upon contact, releasing energy in the form of high-energy photons (gamma rays). This property has led to numerous applications and potential uses of antimatter, including medical imaging, space propulsion, and even as a potential energy source. However, producing and containing antimatter remains a significant challenge due to its highly reactive nature and the current limitations of technology.
One of the key scientific questions surrounding antimatter is the apparent asymmetry between matter and antimatter in the observable universe. The Big Bang theory suggests that both matter and antimatter should have been created in equal amounts during the birth of the universe. However, the universe as we observe it is predominantly composed of matter, with very little antimatter present. This discrepancy is known as the baryon asymmetry problem, and it remains one of the unsolved mysteries of modern physics.
According to the Big Bang theory, the universe began with an extremely hot and dense state, in which particles and antiparticles were spontaneously created and annihilated. At that time, both matter and antimatter should have been produced in equal amounts. However, as the universe expanded and cooled, the rate of particle-antiparticle production decreased, and eventually, the remaining matter and antimatter should have annihilated each other, leaving behind a universe filled with only radiation.
However, our observations of the universe indicate that it is predominantly composed of matter, with very little antimatter present. Stars, galaxies, and even interstellar gas are all made of matter, and there is no evidence of large-scale antimatter structures. This apparent asymmetry between the amounts of matter and antimatter is what is referred to as the baryon asymmetry problem. The term “baryon” refers to a class of particles, which includes protons and neutrons, the building blocks of atomic nuclei.
To explain the baryon asymmetry problem, scientists have proposed various mechanisms that could have led to a slight preference for matter over antimatter in the early universe. These mechanisms must satisfy certain conditions, known as the Sakharov conditions, which were formulated by Russian physicist Andrei Sakharov in 1967. The Sakharov conditions are:
- Baryon number violation: There must be processes that change the net number of baryons, allowing for a difference between the number of particles and antiparticles.
- C and CP violation: There must be a violation of charge conjugation (C) symmetry, which relates particles to their antiparticles, and combined charge conjugation and parity (CP) symmetry, which relates particles to their mirror images.
- Departure from thermal equilibrium: The universe must be in a non-equilibrium state to prevent the complete annihilation of matter and antimatter.
Various theoretical models have been proposed to explain the baryon asymmetry problem, such as baryogenesis, leptogenesis, and electroweak baryogenesis. These models involve processes occurring at very high energies, typically associated with the early universe or unification of the fundamental forces. However, despite significant progress in understanding the possible mechanisms that could generate baryon asymmetry, a definitive explanation for this phenomenon remains elusive.
The baryon asymmetry problem is a crucial topic in the fields of cosmology and particle physics, as it challenges our understanding of the fundamental laws governing the universe. Resolving this problem would provide insights into the early universe’s conditions and the interplay of the fundamental forces and particles that shaped its evolution.
The energy released in matter-antimatter annihilation is incredibly powerful. According to CERN, the European Organization for Nuclear Research, when a particle and its antiparticle meet and annihilate each other, they release energy equivalent to 25 kilotons of TNT per gram of matter (source: CERN). So, the energy released by the annihilation of 1 gram of matter with 1 gram of antimatter (equivalent to 25 kilotons of TNT) could power approximately 2,729 average U.S. homes for one year.
In 1995, CERN became the first laboratory to produce and trap atoms of antihydrogen, the simplest antimatter atom. This groundbreaking achievement has allowed scientists to study the properties of antimatter in greater detail and compare them with those of ordinary matter (source: CERN).
The production of antimatter is currently extremely expensive and inefficient. It is estimated that producing just one gram of positrons would cost around $25 billion, making it one of the most expensive substances on Earth (source: NASA).
Producing antimatter, specifically positrons in this case, is extremely expensive due to several factors:
- Energy requirements: Antimatter production requires a tremendous amount of energy. Particle accelerators, such as those at CERN, are used to create high-energy collisions that generate particles and antiparticles. These machines consume a significant amount of electricity to operate, which adds to the cost of antimatter production.
- Low production efficiency: The process of creating antimatter in particle accelerators is highly inefficient. Only a tiny fraction of the energy input results in the production of antimatter particles. Most of the energy is converted into heat or other forms of radiation, which do not contribute to antimatter production.
- Antimatter isolation and collection: After antimatter particles are created, they need to be separated from other particles and collected. This process is challenging due to the reactive nature of antimatter and the presence of other particles created during high-energy collisions. Sophisticated detectors and magnetic or electric fields are used to isolate and collect antimatter particles, adding to the complexity and cost of the process.
- Storage and containment: As antimatter annihilates upon contact with ordinary matter, it must be stored in a way that prevents any contact with its surroundings. This typically involves the use of magnetic or electric traps that suspend antimatter particles in a vacuum. The development and maintenance of such containment systems are technologically challenging and expensive.
- Limited facilities: There are only a few facilities worldwide, such as CERN, capable of producing antimatter. These facilities are primarily focused on research and not large-scale antimatter production. The limited availability of antimatter production facilities contributes to the high cost and scarcity of the material.
Experts in the field of particle physics continue to study and explore the properties of antimatter. For example, the ALPHA experiment at CERN aims to measure the properties of antihydrogen with unprecedented precision, comparing them to those of hydrogen to test the fundamental symmetries of nature. Dr. Jeffrey Hangst, the spokesperson for the ALPHA collaboration, has stated that “by studying antimatter, we can learn more about the early universe and why there is an asymmetry between matter and antimatter” (source: CERN).
The book “Antimatter” by Frank Close, is a renowned particle physicist. In his book, Close explains the history, properties, and potential applications of antimatter, providing a comprehensive overview of the subject for general readers.
Antimatter is a fascinating and essential aspect of particle physics that has implications for our understanding of the universe and its origins. Researchers at institutions like CERN continue to study antimatter, making significant advancements in the field. While the potential applications of antimatter, such as energy generation and space propulsion, are intriguing, they remain a distant possibility due to the current limitations of technology and the prohibitive cost of antimatter production.